DESIGNING A SUSTAINABLE FUTURE
TABLE OF CONTENTS
-
Overview of the 2030 Challenge
-
Always Start with Passive Strategies
-
Building Massing
-
Designing a More Efficient Building Envelope
-
Air Leakage and Construction Practices
-
Active Strategies
-
Advanced Building Control Systems
-
Energy-Efficient Equipment and Systems
-
Renewable Energy
-
Conclusion
PART 1: OVERVIEW OF THE 2030 CHALLENGE
The 2030 Challenge is a nationwide initiative aimed at promoting industry-wide commitment to prioritizing optimal energy efficiency strategies in buildings while minimizing their environmental impact. This initiative provides a standardized framework for reporting, enabling all design firms to compete in achieving annual energy use reduction targets (in 2023, the goal was an 80% reduction from the baseline).
Since 2006, the American Institute of Architects (AIA) has endorsed the 2030 Challenge and encouraged firms to commit to reducing fossil fuel consumption, greenhouse gas (GHG) emissions, and energy use in their projects. By 2030, the reduction targets will incrementally increase until all participating firms have the experience and knowledge to achieve Net-Zero ratings (zero net emissions) for all projects.
PART 2: ALWAYS START WITH PASSIVE STRATEGIES
Passive strategies (or passive design) are building design methods that leverage local climate and existing conditions to maintain indoor thermal comfort without using energy. These elements utilize available natural conditions to cool, heat, shade, or ventilate spaces—thereby reducing heating and cooling loads.
Designing with passive strategies requires a clear understanding of constraints and responding with design solutions that do not rely on active mechanical systems. Common examples include:
-
Building form and orientation
-
High-performance insulation
-
High-performance glazing
-
Thermal mass
-
Shading systems
The greatest challenge of passive design is that these solutions need to be integrated from the earliest stages of the design process to be effective. Architects who understand the impact of climate on buildings will be better equipped to create energy-efficient and cost-effective structures.
The American Institute of Architects (AIA) provides a "2030 Palette" tool with a list of applicable passive design strategies, along with guidance on suitable building types and climates.
Passive strategies that can be integrated into the initial design phase (depending on whether the climate is predominantly hot or cold) are compiled in in-depth documents such as:
-
Four Passive Strategies for Energy-Efficient Building Systems
-
Comprehensive Guide to High-Performance Building Design
These encompass three main groups:
-
Energy Efficiency
-
Electrification
-
Renewable Energy
PART 3: BUILDING MASSING
Building massing—that is, the shape and orientation of the building—should always be considered first in design, except in renovation projects where the existing form or location cannot be altered.
From an energy efficiency perspective, a compact building mass is the most effective configuration, especially when the glazing area is less than 40% of the total building envelope. However, it's important to note that reducing glazing area also decreases natural daylight, which may increase the need for artificial lighting.
In many cases, cooling loads are not caused by the building envelope but by internal loads, such as:
- Hospitals
- Laboratories
- Buildings with high occupant or equipment density
This is because equipment and people emit more heat than what is transmitted through the building envelope. However, the envelope can still exacerbate internal loads, leading to significant cooling peaks that require higher-capacity mechanical systems.
Effective massing strategies include:
- Prioritize selecting the building mass based on architectural and urban planning considerations. Then, adjust accordingly based on performance analysis.
- Even when the building is primarily influenced by internal loads, using an envelope that is appropriate for the local climate remains essential.
- Once the building mass and envelope are defined, further analysis of additional factors is required to reduce energy use toward Net-Zero emissions.
PART 4: DESIGNING A MORE EFFICIENT BUILDING ENVELOPE
The use of effective insulation materials for roofs, walls, and glazing can significantly reduce a building’s Energy Use Intensity (EUI) — especially in regions with pronounced seasonal temperature variations. A well-designed building envelope also helps lower peak heating and cooling loads, allowing for smaller mechanical systems and reduced capital investment costs.
However, when considering the building as a whole, it's important to understand that improving performance in one component (such as the envelope) may allow for reduced requirements in others, thereby optimizing the overall project.
1. WALL STRATEGIES
R-value: This is the indicator that measures a wall’s thermal insulation performance.
- Low: R-5 to R-13
- Medium: R-14 to R-18
- High: R-19 to R-40
Depending on the building’s scale, function, and performance analysis, the appropriate R-value will be selected. The higher the R-value, the better the insulation.
Classifications:
🔸 Emissivity also affects the amount of heat transferred through walls.
- It refers to the ratio of thermal radiation emitted by a surface compared to an ideal black body (value ranges from 0–1).
- Wall surfaces with low emissivity (e.g., white, highly reflective walls) absorb less heat, making them suitable for hot climates.
- Dark-colored walls may absorb more heat, making them more suitable for cold climates.
🔸 Thermal Mass
- This is the ability of a material to absorb and store heat.▪️ Materials with high thermal mass (such as concrete or brick) help stabilize interior temperatures, especially when outdoor temperatures fluctuate significantly throughout the day.
- In contrast, materials such as wood or thin metal absorb and release heat quickly, making them unsuitable as the primary envelope in areas with high temperature variability.
2. ROOF STRATEGIES
Similar to walls, the R-value of a roof measures the thermal insulation capability of roofing materials.
- The roof is the uppermost insulated surface of a temperature-controlled space, responsible for resisting solar radiation, absorbing heat, and shielding from rain and wind.
- As part of the thermal envelope, the roof plays a significant role in the transfer of heat into and out of the building.
Roof R-value classifications:
- Low: R-9 to R-18
- Medium: R-19 to R-25
- High: R-26 to R-50
Additional considerations:
- Reflective coatings: help reduce heat absorption, thereby lowering cooling loads.
- Green roofs: provide insulation, reduce urban heat island effects, and cool through evapotranspiration.
3. GLAZING STRATEGIES
Glazing refers to the transparent surfaces of the building envelope, such as windows or glass facades. These elements are essential for:
- Providing natural daylight
- Harnessing solar heat
- Creating visual connection for occupants
Common types of glazing:
- Clear glass, tinted glass
- Tempered glass, laminated safety glass
- Glass with special coatings (Low-E, reflective coatings, etc.)
- Can be single, double, or triple glazed units
Key glazing performance metrics:
-
U-value (Thermal Transmittance):
▪️ The lower the value → the better the insulation → reduced heating/cooling loads -
SHGC (Solar Heat Gain Coefficient):
▪️ Ranges from 0 to 1
▪️ Higher SHGC → more solar heat enters → increased cooling load
▪️ In hot climates (ASHRAE zones 1–4), glazing with SHGC < 0.4 is recommended -
VT – Visible Transmittance:
▪️ Indicates the amount of visible light passing through the glass
▪️ Ranges from 0 to 1 (or 0% to 100%)
▪️ Clear glass can reach VT ~90%
▪️ Tinted or coated glass typically ranges from 35%–80% VT
▪️ VT should be adjusted based on window orientation to reduce glare and overheating, e.g., south-facing windows in hot climates -
Shading Devices (Blinds, Curtains, Screens):
▪️ Measured by the Shading Device Factor (SDF)
Example: a blind with SDF = 0.35 → blocks 65% of incoming light
▪️ Light-colored or mesh-type external blinds provide better light control
PART 5: AIR LEAKAGE AND CONSTRUCTION PRACTICES
1. AIR INFILTRATION
Air infiltration refers to the unwanted entry of outdoor air into a building through cracks, gaps, or poorly sealed doors and windows. This phenomenon impacts:
- Indoor Air Quality (IAQ)
- Energy consumption, as HVAC systems must condition unintentional airflow
Air leakage control standards:
- Global standards such as Passive House require infiltration rates ≤ 0.6 ACH @ 50 Pa
- (ACH: air changes per hour at a test pressure of 50 pascals)
Factors affecting air infiltration:
- Airtightness of the building envelope
- Wind speed and direction
- Stack effect
- Pressure differentials between interior and exterior
Controlling air infiltration requires coordination from design through construction to final commissioning.
2. STACK EFFECT AND ITS IMPACT ON AIR LEAKAGE
The stack effect refers to the vertical movement of air within a building caused by temperature differences between the interior and exterior.
- In high-rise buildings, this effect can create zones of negative pressure, drawing in unconditioned outdoor air → causing severe infiltration
- This significantly increases heating/cooling loads, reducing overall building performance
Strategies to control air infiltration:
- Comply with stringent airtightness standards such as Passive House
- Perform preliminary blower door tests to detect and fix leakage points
- Install an effective and continuous air barrier during construction
- Use air locks at main entrances and exits if necessary
PART 6: ACTIVE STRATEGIES
Active strategies refer to design methods that utilize mechanical systems or fuel-based systems to heat, cool, or ventilate spaces. Examples include:
- Electric lighting systems
- Control sensors
- HVAC systems
While passive strategies should be prioritized first to optimize comfort and reduce energy consumption, active strategies are used to meet remaining building requirements when passive measures alone are insufficient.
TECHNOLOGY – THE KEY TO NET-ZERO
Technology is an essential component in the journey toward Net Zero. From electrification to air purification systems, integrating technology into the built environment has unlocked numerous opportunities to enhance energy efficiency.
Many advanced technologies are already available today, but they only deliver optimal results when integrated from the early design phase to ensure that Net Zero goals are upheld throughout the building's lifecycle.
PART 7: ADVANCED BUILDING CONTROL SYSTEMS
Advanced building control systems refer to systems and technologies used to manage, monitor, and optimize the performance of devices and systems within a building.
1. LIGHTING CONTROL
- Lighting accounts for over 19% of global electricity consumption. Using high-efficiency lighting technologies and intelligent control systems such as:
- Dimmers
- Occupancy sensors
- Daylight sensors
- Timers
… can significantly reduce energy use and operational costs.
🔸 Occupancy Sensors
- Devices that detect motion and automatically turn lights, air conditioning, or ventilation systems on or off based on human presence.
🔸 Daylight Sensors
- Devices that measure natural light levels in a space and send signals to the control system → automatically dim or turn off electric lights when there is sufficient daylight.
- This process is called Daylight Harvesting → helping to save 20–60% of lighting energy.
➡ These sensors are essential in Net-Zero building design. If their inclusion in the final design is uncertain, simulations should be conducted to evaluate potential energy savings and the payback period when installing some or all sensors.
2. BUILDING ENERGY MANAGEMENT SYSTEM (BEMS)
Building Automation Systems (BAS) or Building Energy Management Systems (BEMS) are responsible for:
- Collecting data from building devices via sensors, meters, controllers, and actuators
- Analyzing data, detecting faults, and sending alerts to facility managers
- Enabling control and decision-making for efficient and energy-saving building operation
🔸 Benefits:
- Simple BEMS → adjusts operations based on user demand
- Advanced BEMS → optimizes controller operations, manages alerts, monitors performance
- Can reduce overall building energy consumption by 10–30%
PART 8: ENERGY-EFFICIENT EQUIPMENT AND SYSTEMS
1. EQUIPMENT
Energy consumed by appliances used in daily life and operations is referred to as plug loads, also known as miscellaneous loads or auxiliary loads. These include: Computers, Coffee machines, Refrigerators, TVs, and other user-operated electronics.
In addition to directly impacting overall electricity consumption, the heat emitted by these devices increases cooling loads, thus affecting the building’s annual energy use.
🔸 Advantages of equipment:
- Shorter lifespan compared to the building envelope → replaced more frequently (every 5–10 years)
- This provides an opportunity to continuously improve the building’s energy performance.
➡ Choose high-efficiency appliances — prioritize ENERGY STAR certified products or equivalent.
Each building type has its own Equipment Power Density (EPD) depending on its function. You may consult energy-efficient equipment selection guides to get started.
2. HEATING, VENTILATION, AND AIR CONDITIONING (HVAC)
According to the U.S. Department of Energy (US DOE):
- HVAC accounts for 90% of energy consumption in residential buildings
- And 60% in commercial buildings
➡ Choosing the right HVAC system for your design has a direct impact on building efficiency, electricity bills, and user comfort.
🔸 Key considerations:
- The heating/cooling demand of the building
- System efficiency and environmental impact
- Building shape and spatial layout
- Installation and operational challenges
Typically, the selection of HVAC systems is the responsibility of mechanical, electrical, and plumbing (MEP) engineers. However, you may refer to resources such as the “Commercial HVAC System Selection Guide” to better understand and coordinate effectively.
PART 9: RENEWABLE ENERGY
Supplying energy from renewable sources is the final tool to achieve the goal of Net-Zero emissions. This is achieved through on-site electricity generation technologies such as:
- Photovoltaic panels (PV)
- Wind turbines
- Other sources: bioenergy, geothermal, hydrogen, hydropower
🔸 Benefits of renewable energy
- Reduces dependency on the traditional power grid, thereby saving energy costs.
- Lowers greenhouse gas emissions, as renewable sources emit little to no CO₂.
- Helps replace high-emission energy sources such as coal or oil.
PHOTOVOLTAIC SYSTEMS (PV – PHOTOVOLTAICS)
PV (solar panels) is one of the most reliable and widely used methods of renewable energy generation today. Using panels with photovoltaic cells, they:
- Absorb sunlight
- Convert it into clean electricity for immediate use
🔸 Implementing PV in a project:
- The first step is to determine the system size and the percentage of energy it will supply to the building.
- Installation locations:
- On rooftops (as long as not shaded by mechanical equipment)
- On carport structures
- On standalone frames
🔸 Important notes:
- Always optimize energy consumption before installing renewable energy systems.
- Since the initial investment cost for PV systems is relatively high, reducing energy demand beforehand helps decrease the required system size—thereby optimizing both cost and project feasibility.
PART 10: CONCLUSION AND FINAL THOUGHTS
THE FIRST STEP TO ACHIEVE THE 2030 GOALS:
🔸 SET CLEAR GOALS
The very first and most important task to achieve the 2030 Challenge is to clearly define the goals you are committed to pursuing. Plan together with key members of the design team to develop:
- A list of specific tasks to be completed
- A realistic and achievable timeline
🔸 REASONABLE STRATEGY
- Prioritize passive solutions to minimize heating and cooling loads.
- Then integrate active solutions, followed by renewable energy.
- Always verify with simulations to ensure the proposed solutions are truly effective. If results are unusual, it may be due to incorrect or incomplete input assumptions.
Tags
Related news
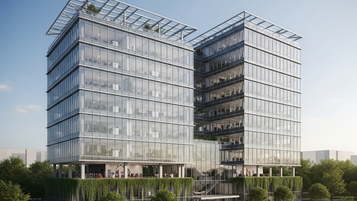
High-Performance Building Design
Part 1: Introduction The term “high performance” can conjure up many images – from an outstanding student, a gifted violinist,...
View detail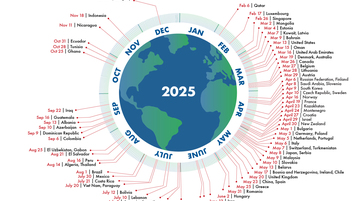
THE JOURNEY TOWARD SUSTAINABLE BUILDING DESIGN
– Insights from a Sustainable Architecture Expert – 🌱 Sustainable design is no longer a choice — it's a responsibility.🧭...
View detail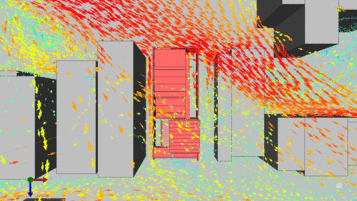
Comparison Table: Natural Ventilation Requirements (ASHRAE, LEED, WELL)
Below is a professional comparison of natural ventilation requirements across three leading standards: LEED v4/v4.1, WELL v2, and ASHRAE 62.1....
View detail